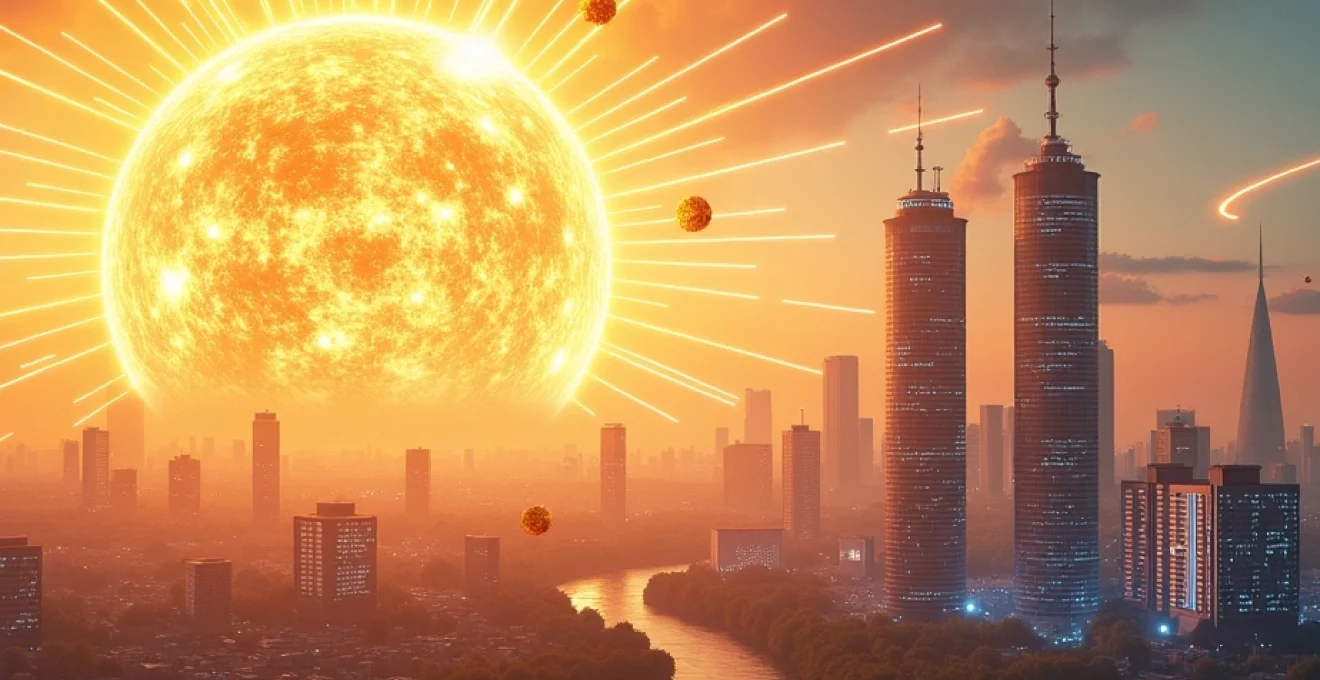
Solar energy represents a pivotal shift in how we power our world, offering a clean, renewable alternative to traditional fossil fuels. As climate change concerns intensify, understanding the intricacies of solar power becomes increasingly crucial. This remarkable technology harnesses the sun's abundant energy, transforming it into electricity that can light homes, power businesses, and drive innovation across industries.
The science behind solar energy is both fascinating and complex, involving cutting-edge materials, advanced engineering, and sophisticated systems that work in harmony to capture and convert sunlight. From the microscopic world of photovoltaic cells to the massive scale of concentrated solar power plants, solar technology continues to evolve, pushing the boundaries of efficiency and applicability.
Photovoltaic cell technology: from silicon to perovskites
At the heart of solar energy conversion lies the photovoltaic (PV) cell, a marvel of modern science that directly converts light into electricity. Traditional PV cells rely on silicon, a semiconductor material that has dominated the solar industry for decades. When photons from sunlight strike a silicon cell, they excite electrons, creating an electric current—a phenomenon known as the photovoltaic effect.
Silicon cells have come a long way since their inception, with researchers continuously refining their design to improve efficiency. Today, high-quality silicon cells can achieve conversion efficiencies of over 20%, a significant improvement from the early days of solar technology. However, the quest for even greater efficiency has led scientists to explore alternative materials.
Enter perovskites, a class of materials that has taken the solar research community by storm. Perovskite solar cells have shown remarkable potential, achieving efficiencies comparable to silicon in a fraction of the time it took silicon to reach those levels. What makes perovskites particularly exciting is their ability to be manufactured using simpler, less energy-intensive processes, potentially lowering the cost of solar panels dramatically.
The rapid advancement in perovskite technology highlights the dynamic nature of solar research. As we push the boundaries of materials science, we inch closer to a future where solar energy could become the dominant power source worldwide.
Solar panel components and efficiency factors
While photovoltaic cells are the cornerstone of solar energy conversion, a complete solar panel system comprises several critical components working in tandem. Understanding these elements and the factors that influence their efficiency is crucial for optimizing solar energy production.
Monocrystalline vs. polycrystalline silicon cells
When it comes to silicon-based solar panels, two main types dominate the market: monocrystalline and polycrystalline. Monocrystalline cells are made from a single crystal structure, offering higher efficiency and a sleek, uniform appearance. Polycrystalline cells, composed of multiple silicon fragments, are less expensive to produce but typically offer slightly lower efficiency.
The choice between mono and poly cells often comes down to a balance of cost, available space, and desired energy output. Monocrystalline panels are ideal for installations where space is limited and maximum efficiency is crucial, while polycrystalline panels offer a more budget-friendly option for larger installations.
Anti-reflective coatings and light trapping techniques
To maximize the amount of light absorbed by solar cells, manufacturers employ various techniques to reduce reflection and trap light within the cell. Anti-reflective coatings, similar to those used on eyeglasses, help to minimize the amount of light that bounces off the surface of the panel. These coatings can increase light absorption by up to 5%, significantly boosting overall panel efficiency.
Light trapping techniques go a step further by manipulating the path of light within the cell. By texturing the surface of the silicon or incorporating specialized structures, engineers can increase the optical path length of light within the cell, enhancing the chances of photon absorption. These advanced techniques can lead to thinner, more efficient solar cells, reducing material costs while improving performance.
Inverter technology: string vs. microinverters
Inverters play a crucial role in solar energy systems, converting the direct current (DC) produced by solar panels into alternating current (AC) used in homes and the electrical grid. Two main types of inverters dominate the market: string inverters and microinverters.
String inverters are the traditional choice, connecting multiple panels in a series and converting their combined DC output to AC. While cost-effective for large installations, string inverters can suffer from reduced efficiency if one panel underperforms due to shading or damage.
Microinverters, on the other hand, are attached to each individual panel, converting DC to AC at the source. This approach allows for optimized performance of each panel independently, mitigating the impact of shading or panel-specific issues. While more expensive upfront, microinverters can lead to higher overall system efficiency and easier maintenance.
Maximum Power Point Tracking (MPPT) systems
Maximum Power Point Tracking (MPPT) systems are sophisticated control mechanisms that ensure solar panels operate at their highest efficiency point. As environmental conditions like temperature and sunlight intensity fluctuate throughout the day, the optimal voltage and current for maximum power output also change.
MPPT systems continuously adjust the electrical operating point of the panels to extract the maximum possible power. By dynamically optimizing this relationship, MPPT can increase energy harvest by 20-30% compared to non-MPPT systems, especially in variable weather conditions.
MPPT technology represents one of the most significant advancements in solar energy efficiency, allowing systems to adapt in real-time to changing environmental conditions and maximize energy production.
Concentrated solar power (CSP) systems
While photovoltaic panels dominate the residential and commercial solar markets, concentrated solar power (CSP) systems offer an alternative approach for large-scale energy production. CSP technology uses mirrors or lenses to concentrate a large area of sunlight onto a small area, generating intense heat that can be used to drive traditional steam turbines or engines to produce electricity.
Parabolic trough collectors: Ivanpah Solar Electric Generating System
One of the most common CSP designs is the parabolic trough collector. These systems use long, curved mirrors to focus sunlight onto a tube filled with a heat-transfer fluid. As the fluid heats up, it's used to generate steam, which then drives a turbine to produce electricity.
The Ivanpah Solar Electric Generating System in California's Mojave Desert is a prime example of large-scale CSP technology. Covering 3,500 acres and boasting a capacity of 392 megawatts, Ivanpah demonstrates the potential of CSP to provide significant amounts of clean energy. However, it also highlights challenges such as water usage in arid regions and the need for vast land areas.
Solar power towers: gemasolar thermosolar plant
Solar power towers represent another innovative approach to CSP. These systems use a field of flat, movable mirrors (heliostats) to concentrate sunlight onto a central receiver at the top of a tower. The Gemasolar Thermosolar Plant in Spain is a pioneering example of this technology, capable of generating power 24 hours a day thanks to its advanced molten salt storage system.
The ability to store thermal energy and generate electricity even when the sun isn't shining gives CSP a significant advantage over traditional PV systems in terms of grid reliability and baseload power generation.
Fresnel reflectors and dish stirling engines
Linear Fresnel reflectors and dish Stirling engines represent two other approaches to CSP technology. Fresnel reflectors use long, thin mirror strips to concentrate sunlight onto elevated receivers, offering a more compact and potentially less expensive alternative to parabolic troughs.
Dish Stirling systems, on the other hand, use a large, dish-shaped concentrator to focus sunlight onto a receiver at the dish's focal point. This receiver is typically coupled with a Stirling engine, which converts heat into mechanical power to drive an electrical generator. While highly efficient, dish Stirling systems are less common due to their complexity and maintenance requirements.
Thermal energy storage: molten salt technology
One of the most significant advantages of CSP systems is their ability to incorporate thermal energy storage, allowing for electricity generation even when the sun isn't shining. Molten salt technology has emerged as a leading solution for this purpose.
In a molten salt storage system, excess heat generated during the day is used to heat a mixture of salts, typically a blend of sodium and potassium nitrates. This molten salt can retain heat for extended periods, allowing the plant to generate electricity during cloudy periods or at night. The Gemasolar plant, for instance, can provide up to 15 hours of electricity generation without sunlight, effectively addressing one of the primary challenges of solar energy: intermittency.
Grid integration and energy storage solutions
As solar energy continues to grow in popularity and scale, integrating this variable energy source into existing power grids presents both challenges and opportunities. Advanced technologies and innovative approaches are being developed to ensure smooth grid integration and to address the intermittent nature of solar power.
Smart inverters for grid stability and voltage regulation
Smart inverters represent a significant advancement in solar grid integration technology. Unlike traditional inverters that simply convert DC to AC, smart inverters can communicate with the grid and adjust their output in real-time to help maintain grid stability.
These advanced inverters can provide crucial grid support functions such as voltage regulation, frequency response, and reactive power control. By actively responding to grid conditions, smart inverters help mitigate some of the challenges associated with high penetrations of solar energy on the grid, such as voltage fluctuations and power quality issues.
Battery technologies: Lithium-Ion vs. flow batteries
Energy storage is crucial for maximizing the potential of solar energy, allowing excess power generated during sunny periods to be used when the sun isn't shining. Lithium-ion batteries, well-known for their use in electric vehicles and consumer electronics, have become a popular choice for solar energy storage due to their high energy density and decreasing costs.
However, flow batteries are emerging as a promising alternative, especially for large-scale, long-duration storage applications. Flow batteries store energy in liquid electrolytes, which can be easily scaled up by simply increasing the size of the storage tanks. While currently more expensive than lithium-ion, flow batteries offer advantages in terms of lifespan, safety, and the ability to provide power for extended periods.
Pumped hydro storage for large-scale energy management
For utility-scale energy storage, pumped hydro remains the most widely used technology globally. In a pumped hydro system, excess electricity is used to pump water uphill to a reservoir. When energy is needed, the water is released back downhill through turbines, generating electricity.
While geographically limited, pumped hydro offers massive storage capacity and long discharge times, making it ideal for balancing large amounts of variable renewable energy on the grid. Innovative designs, such as underground pumped hydro storage, are expanding the potential applications of this technology.
Virtual power plants and distributed energy resources
Virtual Power Plants (VPPs) represent an innovative approach to managing distributed energy resources, including solar panels, batteries, and smart appliances. By aggregating and coordinating these resources, VPPs can provide services typically associated with traditional power plants, such as grid balancing and peak shaving.
VPPs use advanced software and communication technologies to optimize the collective output of distributed resources, enhancing grid stability and maximizing the value of solar and other renewable energy sources. This approach not only improves grid reliability but also creates new opportunities for homeowners and businesses to participate in the energy market.
The integration of smart grid technologies, energy storage solutions, and innovative management approaches is transforming how we think about and utilize solar energy, paving the way for a more flexible, resilient, and sustainable energy system.
Emerging solar technologies and future innovations
The field of solar energy is rapidly evolving, with researchers and engineers constantly pushing the boundaries of what's possible. Emerging technologies and innovative approaches promise to further increase efficiency, reduce costs, and expand the applications of solar power.
Tandem solar cells: improving efficiency limits
Tandem solar cells represent one of the most promising avenues for increasing solar cell efficiency beyond current limitations. By stacking two or more cells made from different materials, each optimized to absorb different parts of the solar spectrum, tandem cells can capture and convert a broader range of light wavelengths.
Recent breakthroughs in perovskite-silicon tandem cells have achieved efficiencies exceeding 29%, surpassing the theoretical limit for single-junction silicon cells. As research progresses, tandem cells could potentially reach efficiencies of 35% or higher, significantly increasing the power output of solar panels.
Organic photovoltaics and flexible solar applications
Organic photovoltaics (OPVs) use carbon-based materials to convert light into electricity. While less efficient than traditional silicon cells, OPVs offer unique advantages such as flexibility, transparency, and the ability to be produced using low-cost, roll-to-roll manufacturing processes.
These characteristics open up new possibilities for solar integration, such as solar-powered clothing, flexible solar panels that can conform to curved surfaces, and semi-transparent solar windows. As efficiency and stability improve, OPVs could find applications in consumer electronics, wearable devices, and building-integrated photovoltaics.
Solar windows and Building-Integrated photovoltaics (BIPV)
Building-Integrated Photovoltaics (BIPV) represent a growing trend in solar technology, where solar cells are seamlessly incorporated into building materials such as windows, facades, and roofing tiles. This approach not only generates electricity but also serves as a functional part of the building envelope.
Transparent solar cells, which allow visible light to pass through while capturing ultraviolet and infrared light, are particularly exciting for BIPV applications. These cells could transform windows into power-generating surfaces without significantly altering their appearance or light transmission properties. As BIPV technologies advance, we may see a future where every surface of a building contributes to its energy production.
Space-based solar power: concept and challenges
Space-based solar power (SBSP) is a visionary concept that involves collecting solar energy in space and beaming it back to Earth. The idea has several potential advantages: solar panels in space would receive sunlight 24/7, unaffected by weather or day-night cycles, and could capture more intense solar radiation due to the lack of atmospheric interference.
However, SBSP faces significant technical and economic challenges. These include the cost of launching and maintaining space-based solar arrays, the efficiency of power transmission from space to Earth (typically proposed using microwave or laser beams), and potential environmental and safety concerns associated with power beaming.
Despite these challenges, several space agencies and private companies are exploring the feasibility of SBSP. As launch costs decrease and solar and wireless power transmission technologies improve, space-based solar power may become a reality in the coming decades, potentially providing a constant, clean energy source on a global scale.